Dental caries remains the most prevalent chronic disease in both children and adults, imposing significant financial and societal burdens on families, governments, and health care systems [1-3]. Among 5-17 year olds, tooth decay is more common than asthma and is responsible for many missed from school and work [1-3]. By age 19, 68% of youth have experienced tooth decay in permanent teeth [4]. Similar trends are observed in adults; 92% of adults 20 to 64 have had dental caries in their permanent teeth [5]. The prevalence of caries suggests that the current standard of care in Dentistry is not effective. Restoration does not treat the underlying disease process; it merely rebuilds the tooth’s shape and for. Therefore Dentistry is undergoing a paradigm shift away from surgical treatment of caries to a model of early caries diagnosis and management. Early noninvasive caries detection will shift dentists’ traditional reactive approach—drilling and filling—to preventive dentistry. However, clinical detection tools and techniques such as x-ray and visual/tactile inspection are not sensitive enough to detect early decay or monitor its progression [6]. For this paradigm to be successful, the development of noninvasive caries imaging technologies is crucial. Detecting early pit and fissure caries is challenging. Radiographic imaging is of minimal diagnostic value because of the large amounts of surrounding enamel [7-8] as it cannot detect small lesions on the order of 50 – 500 μm in the interproximal areas. Those could remineralize if detected early and suitable preventive measures instituted [9]. Studies [10-12] have suggested that dental x-rays are of little help in the detection of occlusal surface caries, and that dental explorers are inefficient for the diagnosis of occlusal caries [13,14]. There is a clear need for more sensitive methods, especially if one wants to practice minimal intervention dentistry or attempt remineralization of carious lesions.
PT methods feature background signal suppression and high sensitivity to subsurface optical absorbers (thermal-wave sources), thereby exhibiting high contrast [14] when using an infrared (IR) camera as the detector, also known as lock-in thermography (LIT) [15]. However, thermal-wave fields, limited by the physics of parabolic diffusion, can only produce thermal-gradient-driven energy transport and are, therefore, lossy or diffuse. As a result, PT techniques have been unable to generate three-dimensional (3D) subsurface imaging, yielding instead depth-integrated images limited in axial resolution by the thermal diffusion length. Tabatabaei and Mandelis [16] introduced a new thermophotonic imaging method enabling parabolic thermal-wave fields to exhibit energy localization akin to propagating hyperbolic wave-fields (e.g. ultrasound). When used with a mid-IR camera, the new approach results in depth-selective (or depth-resolved) thermophotonic imaging. It greatly improves axial and depth resolution and allows for individual image slices of axially superposed subsurface distributed absorbers/sources using novel image generation and processing waveforms based on radar principles. The result was the birth of a new imaging field, Photothermal Coherence Tomography (PCT) [17] which is based on the concept of the Thermal-Wave Radar (TWR) which was pioneered in the CADIPT.
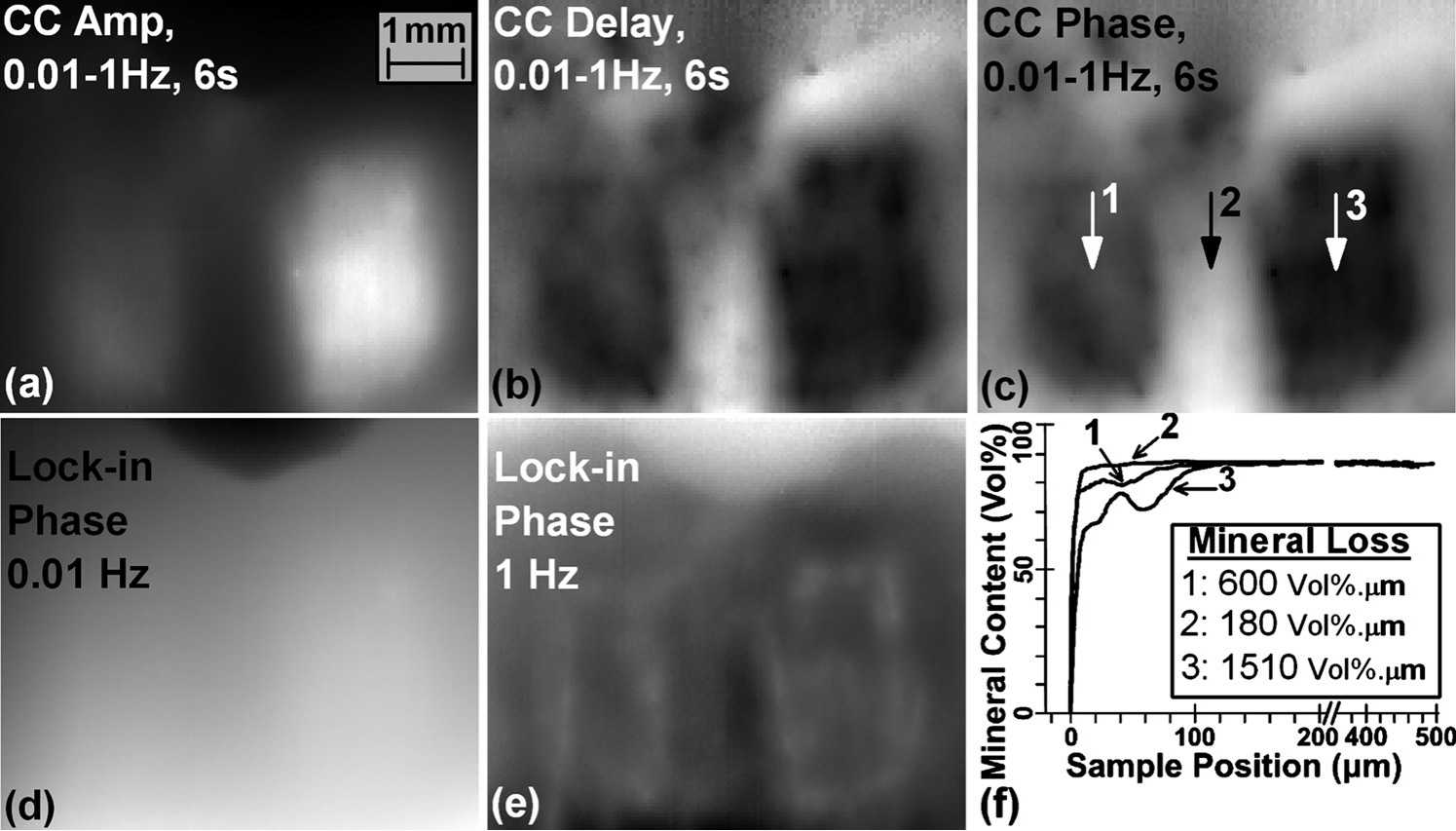
Thermophotonic radar and phase lock-in imaging of a tooth with 10-day (left) and 20-day (right) enamel demineralization treatment demineralized using an acidic gel within two square-shaped treatment windows. CC: Cross-correlation; CC delay: peak delay time image. Chirp parameters: 0.01–1 Hz in 6 s. LIT phase images at (d) 0.01 Hz and (e) 1 Hz; (f) Transverse Micro-Radiography (TMR) mineral profiles at points 1–3 indicated in (c), the inset depicts the amount of mineral loss [17].
References I.6
[1] U.S. Department of Health and Human Services, Oral Health in America: A Report of the Surgeon General, National Institute of Dental and Craniofacial Research, 2000.
[2] Benjamin RM, Oral Health The Silent Epidemic Surgeon General’s Perspective, Public Health Rep, 2010; 125:158 – 159.
[3] Abanto J, Caravalho TS, Mendes FM, Wanderley MT, Bönecker M, Raggio DP, Impact of oral diseases and disorders on oral health-related quality of life of preschool children, Community Dent Oral Epidemiol, 2011; 39(2):105-114.
[4] U.S. Department of Health and Human Services, Healthy People 2010 Final Review, Centers for Disease Control and Prevention, 2012. URL: http://www.cdc.gov/nchs/healthy_people/hp2010.htm
[5] U.S. National Institute of Dental and Craniofacial Research, Report on the National Health and Nutrition Examination Survey 2004. URL: http://www.nidcr.nih.gov/DataStatistics/FindDataByTopic/DentalCaries/DentalCariesAdults20to64.htm
[6] National Institutes of Health Consensus Development Conference Statement, Diagnosis and Management of Dental Caries throughout Life, J Dent Educ, 2001; 65(10): 1162–8.
[7] McKnight-Hanes C, Myers DR, Dushku JC, Thompson WO, Durham LC. Radiographic recommendations for the primary dentition: comparison of general dentists and pediatric dentists. Pediatr Dent, 1990;12(4):212-216.
[8] Flaitz CM, Hicks MJ, Silverston LM. Radiographic, histologic, and electronic comparison of basic mode videoprints with bitewing radiography. Caries Res, 1993; 27(1): 65-70.
[9] Backer DO, Post-eruptive changes in dental enamel, J Dent Res 1966; 45: 503-551.
[10] Hintze H, Wenzel A, Clinically undetected dental caries assessed by bitewing screening in children with little caries experience, Dentomaxillofac Radiol 1994; 23(1): 19-23.
[11] Nytun RB, Raadal M, Espelid J, Diagnosis of dentin involvement in occlusal caries based on visual and radiographic examination of the teeth, Scand J Dent Res 1992; 100(3): 144-148
[12] Flaitz CM, Hicks MJ, Silverstone LM, “Radiographs, histologic and electronic comparison of occlusal caries: An in vitro study” Paediatr Dent, 1986; 8(1): 24-28.
[13] Penning C, van Amerongen JP, et al, Validity of probing for fissure caries diagnosis. Caries Res, 1992; 26:445-449.
[14] Almond DP, Patel P.M. Photothermal Science and Techniques; 1996.
[15] Maldague XP. Theory and Practice of Infrared Technology for Nondestructive Testing. New York: Willey; 2001.
[16] Tabatabaei N, Mandelis A. Thermal coherence tomography using match filter binary phase coded diffusion waves. Phys Rev Lett, 2011;107(16):165901.
[17] Tabatabaei N, Mandelis A, and Amaechi B, Thermophotonic radar imaging; An emissivity-normalized modality with advantages over phase lock-in thermography, Appl. Phys. Lett. 98, 163706 (1 – 3) (2011).