The non-invasive measurement of blood glucose has long been a “holy grail” in biosensor science and technology [1]. For more than 20 years, scientists have tried to use beams of near-infrared light to measure glucose levels in the body. This light passes through human tissue harmlessly. Chemical bonds in the glucose molecule (the pyrene ring) vibrate when struck by it, absorbing energy. This absorption can be measured, providing information about the concentration of glucose in the blood. But this fingerprint of glucose is easily smudged. Other materials floating around in the blood can also absorb near-infrared radiation, creating a mess that’s difficult to untangle. For a more specific signature, some researchers have turned to slightly longer wavelengths. Mid-infrared light photons are absorbed by glucose, but not by other molecules in the blood — with one huge exception. Water, which makes up most of the blood volume, overwhelmingly soaks up mid-IR photons. The challenge has been to overcome the absorption barrier of water. To erase this background interference, wavelength-modulated differential photothermal radiometry (WM-DPTR) was pioneered in the CADIPT. WM-DPTR is a sensitive [2,3], patented [4] non-invasive and non-contacting technique for measuring blood sugar indirectly in the interstitial fluid. It uses a pair of laser beams instead of a needle. One is absorbed by both water and glucose, the other only by water. When both beams strike dissolved glucose coherently (out-of-phase operation), the water absorptions cancel each other out, giving off measurable heat that highlights just the glucose. Tested on glucose dissolved in water and on human blood serum, the technique has proved to be sensitive even to small quantities of glucose. The glucose sensitivity depends strongly on the combination of two parameters: the intensity ratio, R, of, and the modulation phase shift, dP, between, the two laser beams. Sensitive and repeatable glucose measurements require tight control of R and dP. Table 1 shows proof of the superior performance of WM-DPTR to other non-invasive glucose diagnostic methods reported to-date.
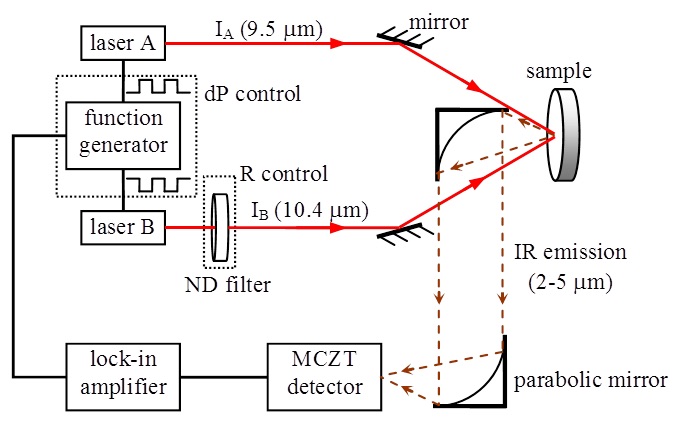
WM-DPTR set-up
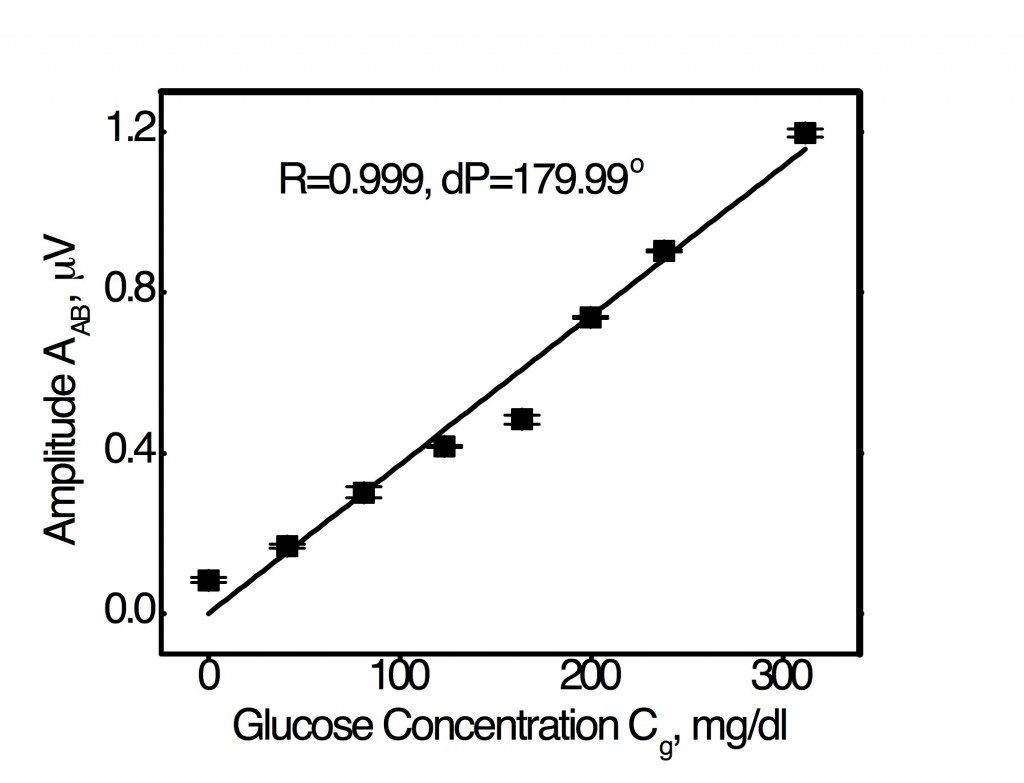
Optimal R-dP combination for linear WM-DPTR signal amplitude response to glucose in serum across the entire physiological glucose concentration range from 20 mg/dl to 320 mg/dl.
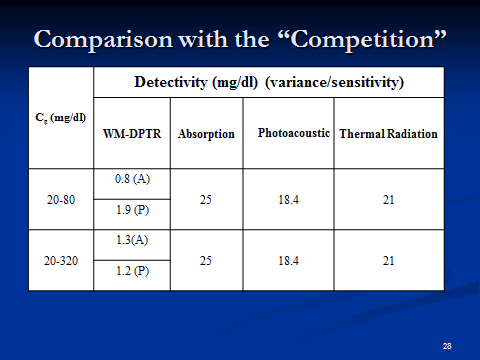
Table 1
The optimal R-dP combination figure shows an extraordinary and unique feature of WM-DPTR technology for detecting glucose in human blood serum: Glucose concentration detection sensitivity with judiciously chosen R and dP values can be a (essentially) linear function of signal output which uses an averaged sensitivity across the entire concentration range, or it can be tuned to higher sensitivities in the hypoglycemic range (not shown) by adjusting the R and dP values. The error bars on the data points are the results of standard deviations from five sequential measurements. These measurement variances are well below the evaluation criteria for a SMBG device set by the International Organization for Standardization (15 mg/dl for Cg < 75 mg/dl and 20% for Cg > 75 mg/dl) and by the Clarke-EGA Error Grid analysis for blood glucose monitoring (< 70 mg/dl for values < 70 mg/dl, and 20% for values > 70 mg/dl) [5], accepted as one of the “gold standards” for determining the accuracy of blood glucose meters. WM-DPTR exhibits clearly superior performance across the human blood glucose concentration. It exhibits unprecedented performance in the hypoglycemic range which is clinically most demanding and where state-of-the-art glucose meter accuracy is the common problem in the SMBG evaluation of state-of-the-art devices.
Currently, the WM-DPTR method is also expanding its applications to the measurement of blood alcohol in the interstitial fluid [6].
References I.7
[1]Smith J.L., The Pursuit of Noninvasive Glucose: “Hunting the Deceitful Turkey, Copyright 2006 by John L. Smith. URL http://www.mendosa.com/ noninvasive_glucose.pdf
[2] Mandelis A, and Guo X, Wavelength modulated differential photothermal radiometry: theory and experimental applications to glucose detection in water, Physical Review E, 84, 041917 (2011).
[3] Guo X, Mandelis A, and Zinman B, Non-invasive glucose detection in human skin using wavelength modulated differential laser photothermal radiometry, Biomed. Opt. Express 3 (11) 3012–3021 (November 2012).
[4] “Method of performing wavelength modulated differential photothermal radiometry with high sensitivity”, Inventors: Andreas Mandelis and Xinxin Guo, US patent 8,649,835 B2, issued February 11, 2014.
[5] Clarke WL, Cox D, Gonder-Frederick LA, Carter W, and Pohl SL: Evaluating clinical accuracy of systems for self-monitoring of blood glucose, Diabetes Care 10, 622 (1987).
[6] Guo X, Mandelis A, Liu YJ, Chen B, Zhou Q, and Comeau F, Noninvasive in-vehicle alcohol detection with Wavelength-Modulated Differential Photothermal Radiometry, Biomed. Optics Express 5 (7)2333-2340 (June 18, 2014)DOI:10.1364/BOE.5.002333.